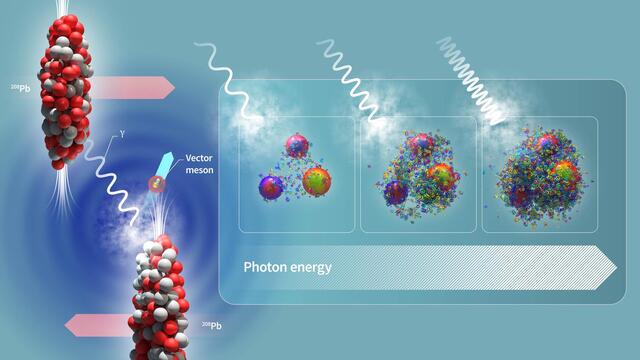
Coverage in home.cern
The CERN Large Hadron Collider (LHC) is not only the highest energy collider of protons and nuclei in the world, it also produces the highest energy photons available in the laboratory. An ultra-relativistic electromagnetically charged projectile such as the proton or lead beams at the LHC carries a strongly contracted field that can be a source of photons. In ultra-peripheral collisions (UPC), the two beams do not hit head-on, but one of the beams emits a photon of very high energy that strikes the other beam, giving rise to photon-proton, photon-nucleus and even photon-photon collisions. These collisions allow scientists to use light with very short wavelengths to reveal the inner structure of the beam particles.
Shining light to the proton
The interactions of high-energy photons with the colliding partner, referred to as the “target”, provide high-resolution pictures of the inner structure of the proton or nuclei. While we know that the proton—and most of the visible matter of the Universe—is made of gluons, the theory that describes quarks and gluons – quantum chromodynamics (QCD) – has not yet provided a complete understanding of the rich physics phenomena that occur in high energy interactions involving hadrons. For example, while gluons are responsible for gluing together the quarks to form protons, at high energies it is predicted that a new state of matter could be formed, the "Color Glass Condensate”. Gluons can interact among themselves in complex ways, scattering off each other, splitting, and recombining themselves. For very high energy interactions such as a “dance of gluons” within the proton reaches a dynamical equilibrium that physicists call “gluon saturation”. So far, it has turned out to be challenging to experimentally measure this QCD phenomenon. The ALICE Collaboration has published new experimental analyses using UPCs, pushing forward our understanding of gluonic saturated matter at the LHC.
Probing gluons within nuclei at high energies
Theory predicts that the inner structure of hadrons will be different as energy increases and that the gluon saturation phenomenon can occur for heavy nuclei at lower energies compared to that of protons. For these reasons, the ALICE Collaboration has studied the energy dependence of UPC processes for both protons and heavy nuclei. At the same time, it is well-known that in nuclei there are other physics phenomena such as gluon shadowing – originating from multi-scattering processes, that can have similar experimental signatures like gluon saturation. The interplay between these two phenomena is still an open problem in the field of QCD interactions. ALICE has published new results on coherent J/ψ mesons—cases when the photon probes the whole nucleus. The new ALICE results probe a wide range of photon-nucleus collision energies from around 10 GeV to 1000 GeV. The new results confirm previous measurements by ALICE, obtained at lower energies, showing a strong “shadowing factor” that compares lead target results with those from protons, and extend the measurement to larger energy. At present the data at high energies can be described by saturation-based models, but also by gluon shadowing models. None of the available theoretical models can fully describe the energy dependence of this process over the explored energies.
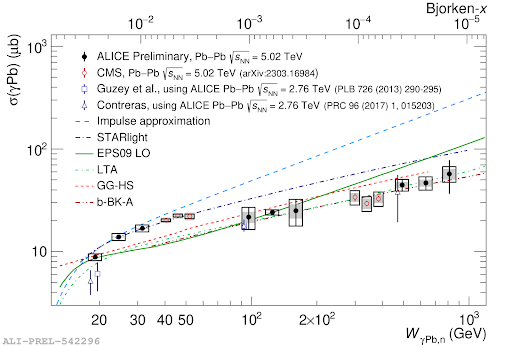
Energy dependence of the coherent J/ψ photonuclear cross section to probe gluon dynamics within nuclei over an extended kinematic region. Both gluon saturation and shadowing models give a reasonable description of data, while the calculation without saturation (blue dashed line) does not.
Gluonic hotspots
The inner structure of protons and nuclei is not static, and there are sub-nucleonic quantum fluctuations within the proton. If gluon saturation occurs, physicists expect that such fluctuations would be very large. The ALICE Collaboration has provided two new measurements that are sensitive to gluonic hotspots. For proton targets, ALICE data show for the first time that it is possible to study different geometrical configurations of the quantum fluctuations by measuring a process where the proton dissociates. This measurement is particularly challenging at hadronic colliders, and the new data represent the first step towards studying the energy evolution of this process. In addition, for lead targets, the ALICE Collaboration presented new results of J/ψ production in UPCs. This study was carried out for “incoherent” reactions where the photon interacts with a single nucleon in the nucleus. The measurement consisted in studying the momentum transferred to the target during the photon-proton interactions. The ALICE data can only be described when the sub-nucleonic fluctuations are considered in the theoretical models, confirming the importance of gluonic hotspots at high energies.
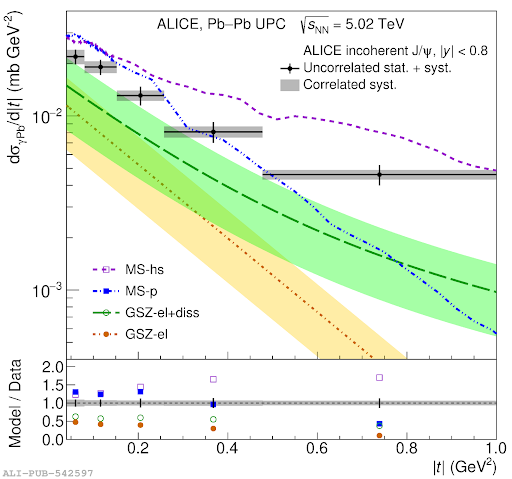
The cross-section as a function of the transferred momentum (t) in incoherent UPC events, where a photon emitted by a lead nucleus from one of the beams interacts with a single proton or neutron and produces a J/ψ particle. The data can be described by theoretical models that include sub-nucleonic quantum fluctuations.
J/ψ polarization
Furthermore, new results on the polarization of J/ψ were also presented. The ALICE data confirm that the helicity of the photon is transferred to the polarization of the J/ψ. Although this was expected by theory, such a measurement confirms that ALICE has now established a detailed scientific program of the measurement of UPC J/ψ and other excited states of particles formed by heavy quarks.
Further reading:
- ALICE Collaboration, 2023 https://arxiv.org/abs/2305.06169
- ALICE Collaboration, 2023 https://arxiv.org/abs/2304.12403
- ALICE Collaboration, 2023 https://arxiv.org/abs/2304.10928
- ALICE Collaboration, 2023 https://arxiv.org/abs/1809.03235
- https://alice-collaboration.web.cern.ch/LHCP2023